What is all the noise about phosphorus these days? This idea that plants do not need the levels of phosphorus that are generally recommended? The amounts recommended by agronomists and plant physiologists are accurate; the problem comes in the interpretations made by the marketing departments of some companies or in the minds of certain self-proclaimed experts.
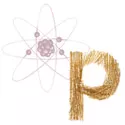
Nutrient recommendations are made with numerous variables in mind, including the composition of the medium, plant variety, pH, temperature, moisture, nutrient interactions, plant requirements, economics, and so on. These recommendations are not just plucked out of the air, and nor are they based on a lay person’s understanding of karmic forces or scientific data.
Beginning look
The best place to begin understanding the phosphorus needs of a plant is with the basics, and the basics include many processes as well as other nutritive elements. Firstly, we need to understand a few basic relationships. Each element has its own weight, which is different from the weight of all the others: one atom of nitrogen weighs less than 1 atom of oxygen which weighs less than one atom of magnesium which weighs less than one atom of phosphorous and so on. Molecules are combinations of atoms which are expressed as the combined weight of all the elements in the molecule. Fertility components may be based on pure elements (based on a pure form of a nutrient, such as Calcium) or compounds (based on a combination of atoms, such as Nitrates, Sulphates, or Phosphates). This is how the plant takes up the nutrient components. This may also be how these are reported on labels or in scientific reports. Few, if any, nutritional elements are taken up by the plant as they are applied: they must either change form, change ionisation properties, or disassociate. This is especially true of phosphorus since it requires a special pathway (known as an H+-HPO4 2- symporter) that takes it up as a phosphate ion after activation.
All the nutritional components applied are subject to competition in the root zone, not only from the plant but also the environment through factors such as temperature, pH, interaction with other elements, and other life forms. Understanding how measurements and samples are taken determines how data sets must be interpreted. Most elements are more concentrated in certain areas of the plant based on the plant itself: for example, leaf tissue (mesophyll) will have as much iron and manganese as it does sulphur and magnesium. Phosphorus, meanwhile, is present in larger amounts in roots and flower tissues (especially seeds). The only way to obtain a complete picture of the composition of the plant is to analyse the entire plant, roots, stems, leaves, shoots, flowers and seeds.
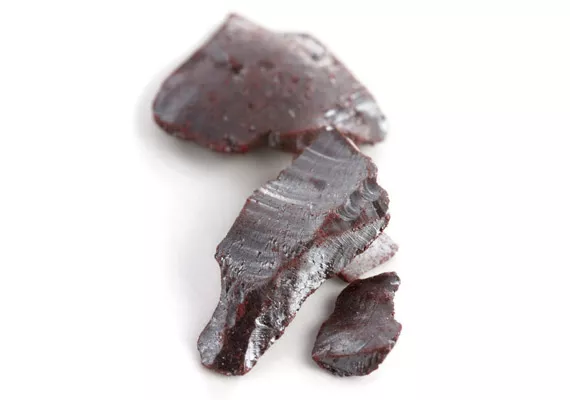
Important estimates can be made, however, by looking at specific tissue analysis, and individual metabolites are usually sought in those areas where they concentrate. Even the way that components are gathered, the time spent in storage or transport, the extraction method used, or the machines used to analyse them, will vary greatly with certain methods being more accurate than others. Sample size is critical in the statistical analysis of the results; the more plants or repetitions in the data set, the more accurate the statements about those data sets are. Accurate results in the data and the interpretation of the data depend on a clear understanding of all the elements involved in the sample; compare Granny Smith apples to Granny Smith apples, not Granny Smith apples to Macintosh apples. Know what you are looking for: looking for sharks in the desert may reveal a few leftovers from a time past when the dessert was a sea, but will not answer the question of how many sharks currently inhabit the planet.
Phosphorus is used by the plant to produce compounds such as sugar phosphates (which is used to store and transfer energy), nucleic acids, nucleotides, coenzymes, phospholipids (membranes), phytic acid, and high energy phosphate bonds (ADP, ATP). The main entry point into the assimilation pathways of phosphate occurs during the formation of ATP (adenosine triphosphate), which acts as the energy currency of the cell.
ATP provides the energy for almost every process in the plant, from uptake of nutrients, and the conversion of nutrient complexes such as nitrates to release the nitrogen that they contain, through to production of DNA and cell division. Photosynthesis is a well-known general process that produces ATP through a process known as photophosphorylation. Respiration is a process that produces ATP through an oxidative process known as O oxidative phosphorylation. Power used in homes and industry is measured in Watts which gives a value for the amount of energy needed to make things work; ATP is used by biochemists to indicate the energy needed to make biological processes occur.
The phosphate group is energy that, once incorporated into ATP, can be converted to energy or transferred by many different processes to form all the phosphorylated compounds present in a plant. These groups may also form other energetic compounds that function in the same basic way as specific processes. The entire pathway and its many routes are known as phosphate assimilation. Phosphate is required to transport most elements into the roots, through cell membranes, and to change nutrients into usable forms; without it, the plant would starve, or rather, cease to grow or function.
Many different elements go to make up plant tissues. Some elements like sodium are specific to certain plants, such as cacti and grasses, while others such as nitrogen, carbon, phosphorous and potassium, are required by all life forms. Concentrations of elements in plant tissues are expressed in terms of ‘Adequate Levels’, which means that enough ions of an element are present to ensure availability when the element is needed for the many processes and metabolites present in plants. When the levels of certain elements are too high, especially nitrogen and heavy metals, this can cause problems, sometimes to the plant itself but usually to animals and life forms that feed on the plant. Table 1 gives a general but fairly accurate idea of the elements needed and the concentration that they should be used in. The table shows that while some elements are equal in terms of percentage composition, there are differences in the actual number of atoms. This relates to the first point made here that each atom has its own unique mass – they do not all weigh the same. Hydrogen, carbon and oxygen are considered critical nutrient elements for the plant but are obtained through water or the air and so are not applicable to applied fertilisers.
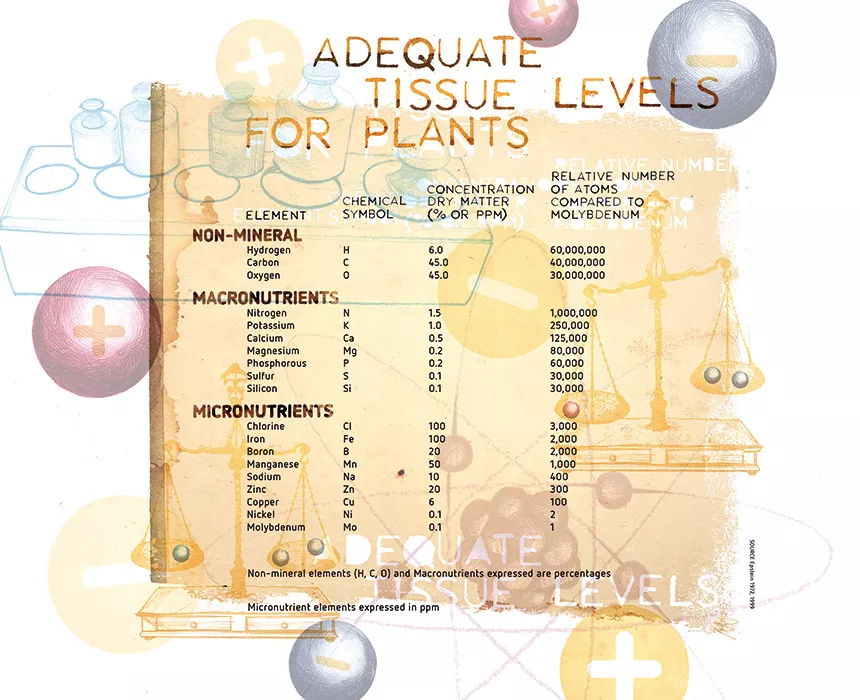
Label discussion
The next critical aspect of this discussion concerns labels: how to interpret them and what they mean. There are as many fertiliser label requirements as there are countries in the world and, in the United States, as many states. Labels are used to show the grower the contents of the nutrients and other constituents of a mixture, slurry, or homogenous blend of nutrient or nutrients. A general discussion of labels used around the world is not the goal of this article; North American markets will be used solely in this discussion. The reader must be aware of the differences across all markets and adapt this knowledge to each different situation. In most cases, these labels are legally acceptable, but not necessarily scientifically acceptable, and sometimes they are based on archaic methods of measuring and, in the case of phosphorus, based on the by-products of burning the compound in enriched air. Science, unhindered by politics, seeks to come as close as possible to an accurate reflection of true events. There are several accurate methods of representing the content of these fertilisers, and several legally acceptable methods. These are mass/mass (m/m) or mass/volume (m/v); in North America, and most other countries around the world now, this is done mass/mass or grams of element per kilogram of fertiliser. The other method is mass/volume or grams/litre.
On all North American and European labels that are registered, elements are given as a percentage of composition, in terms of weight: for every kilogram (or pound) of fertiliser, there is X% by weight of a particular nutritive element. In general, the most prominent or first three numbers to appear on the label represent nitrogen – phosphorous – potassium (N – P – K): for example 10 – 10 – 10. The additional elements may be listed under the guaranteed analysis section of the label, provided the company wants to guarantee those elements in those proportions. N – P – K elements are macronutrients and considered major elements, but macronutrients also include other elements (see Table 1).
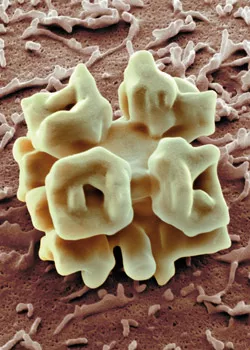
Currently, nutritional elements are classified as either macro or micro elements based on the relative amounts of the component used by the plant. The term ‘component’ is used deliberately here because it may be a compound that is measured rather than a single element; for example phosphorus (P) is measured as phosphate pentoxide (P2O5), and potassium (K) is measured as potassium oxide (K2O). This means that the percentage weight refers not only to that particular element but includes the additional elements in the compound, in this case oxygen (O). Nitrogen (N), on the other hand, is listed as just the atom ‘N’, but the Guaranteed Analysis section will state what this nitrogen is derived from and give this as a percentage of the nitrogen component derived. Different forms of nitrogen act differently and have different properties. So while the percentages are listed correctly on the label, the reality is more complex and a calculation is needed to ascertain the actual amount being applied. When two or three parts are used, such as with some liquid fertilisers, add the similar element numbers together to calculate the correct concentration.
Here is an example for the calculations needed to determine the actual concentrations of nutritive elements taken from North American or European labels where the percentages listed are mass/mass.
A 50-pound container of liquid-based fertiliser with the N – P – K values 10 – 20 – 10 (which, you might assume, implies a 1:2:1 ratio) include the following additional information in the Guaranteed Analysis section:
- Total Nitrogen (N)……………………..10%
- 10% Nitrate Nitrogen
- Available Phosphate (P2O5)………..20%
- Soluble Potash (K2O).…….…………..10%
This means that 10% of 50 pounds (22.6796 kg), i.e. 5 pounds (2.268 kg), is elemental N since it is listed as N not as a compound that contains N; 20% of 50 pounds, or 10 pounds (4.5359 kg) is P2O5; and 10% of 50 pounds, or 5 pounds (2.268), is K2O.
These are the commercial percentages listed on the fertiliser packaging. However, to derive the elemental percentages, a conversion is required since both the K and P weights include oxides. In this example, the percentage of actual P in the oxide form P2O5 is 44% and the percent K is 83%, so the actual weight of elemental P is 4.4 pounds (10 x 0.44) and the weight of K is 4.15 pounds (5 x 0.83) (P is 1.9958 kg and K is 1.8824 kg). So the corrected numbers would read 10% – 8.8% - 8.3%.
So the actual ratio in the fertiliser of single elements in this example is 1.0:0.88:0.83 N – P – K, not the 1:2:1 that the label implies. All other elements given, whether they are actually taken up as a complex like sulphates, or in elemental form, are expressed on the label as the elemental version like nitrogen.
Using different measures of mass/volume, the numbers would be different and are also based on specific gravities. An example would be a Root/Flower additive fertiliser for which the North American mass/mass convention would show a 0 – 10 – 11 NPK value, but the mass/volume percentages may be expressed as 0 – 13 – 14, depending on the compounds used in the fertiliser. The ratio is what is truly important, how much of each element is provided; using higher or lower numbers is relevant to the amount applied, provided the ratio is close. Each species or, sometimes variety of plant, has a ratio specific for its needs even though many plants have identical needs and are sometimes grouped according to these needs. So given three different fertilisers labelled 0 – 10 – 11, 0 – 20 – 22 and 0 – 30 – 33, the ratio stays close and only the amount applied needs to be adjusted on the basis of the requirements of the crop. This is because, in the end, the root zone needs to have a certain amount of nutrients available for the plant when the plant needs to take it up, but many variables can and will affect this requirement as a nutrient moves from the bottle or bag to the utilisation sites in the plant.
Limiting values
Limiting values are the speed limits on the growth and development of a plant or any other life form. These limits are determined by a range of factors, from carbon dioxide (CO2) in the air, to water in the soil, or the availability of a single element. All these factors limit availability, which determines the potential of the plant’s development. They are known as limiting agents, perfect ratios and the amounts of fertiliser can be applied to a plant, but if the available carbon (C) is limited by a lack of CO2 in the air, the plant will not be able to utilise all the nutrients applied and nor can structural elements develop or other processes occur, and the plant will fails: the limiter is carbon.
In any growing system, the goal is to ensure that adequate levels of all the input components are maintained over time and adjusted when necessary because a plant requires different levels of particular elements at different stages in its development. Most nutritive elements should, as mentioned, be kept close to the required levels, rather than exceeding them, because they tend to accumulate in the tissues of the plant where they can become toxic to the plant or to animals that consume it. The ratio in the root zone closely matches overall composition of the plant tissue; it is the concentration that causes problems with salt burns. Also, other factors can greatly influence nutrient availability to the plant, such as pH or substrate composition and nutrient formulation. It does no good to apply the correct ratio of NPK if the pH is out of the correct range since these nutrients will be made more or less available to the plant and will express this difference in tissue composition.
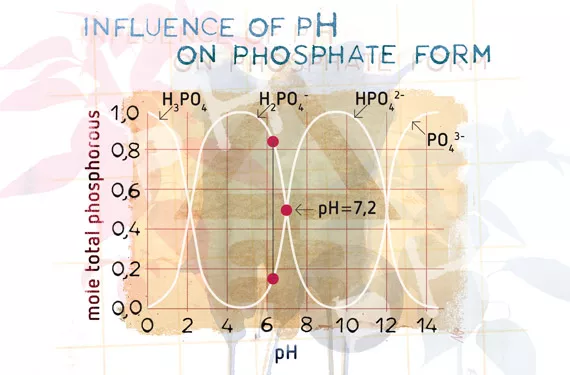
There are many ways of engineering a fertiliser; for instance, nitrogen can be applied as ammonium nitrate, potassium nitrate, calcium nitrate, urea, and so on, but each form is different and each brings other components along with it. Phosphorous can be applied as superphosphate, triple superphosphate, mono-potassium phosphate, ammonium phosphate, or bone meal, to name but a few. Each of these substances needs to be ‘activated’, broken down or form shifted (ionisation) in the root zone, so that it can be taken up in one of the three forms of phosphate that can be taken up by a plant. The pH of the environment will affect the form that the phosphates take and can ultimately limit the availability of the desired monovalent form H2PO4 - at normal pH ranges between 5.2 and 7.2 by converting the phosphates into the unusable form H3PO4 or the less desired divalent form HPO4 2- (see Figure 3)
The phosphates will also bind other available elements as well as to substrate particles and become unavailable to the plant even though they are still detectable in the system. So fertilisers must be designed not only to provide the right ratios of elements in the right amounts, but also to be compatible with a dynamic environment in terms of temperature, pH fluctuations and different substrates.